
Research
Research List
Quantum information technology using photons
Linear optical quantum circuit
A quantum bit (qubit) of a single photon can be precisely controlled with existing devices. Its robustness against decoherence is also a great advantage of using photons. Photons, however, only very weakly interact with each other. This character had prevented us from the realization of controlled NOT (C-NOT) gate of photonic qubits, which is indispensable in quantum computations.
All kinds of the quantum gates, including C-NOT gate, can be constructed with conventional linear optics using two-photon interference. On the basis of these suggestions, we have newly developed Partially Polarizing Beam Splitters (PPBSs) in order to realize C-NOT gate with a smaller number of optical elements. Our PPBS-based C-NOT gate indeed showed excellent stability of the operation, which leads to the realization of quantum computer [1,2].
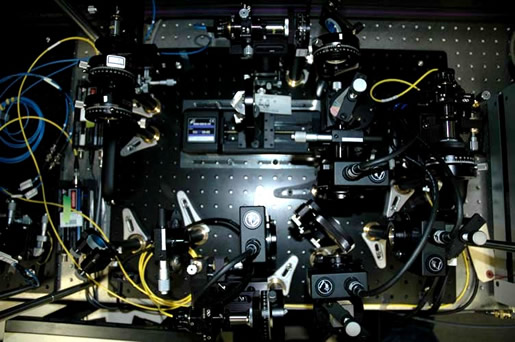
Fig. 1: Experimental setup of our C-NOT gate
[1] H. F. Hofmann and S. Takeuchi, “Quantum phase gate for photonic qubits using only beamsplitters and postselection”, Phys. Rev. A. 66, 024308/1-024308/3 (2002).
[2] R. Okamoto, H. F. Hofmann, S. Takeuchi and K. Sasaki, “Demonstration of an optical quantum controlled-NOT gate without path interference”, Physical Review Letters, 95, 210506/1-4 (2005)
Quantum metrology
Phase measurement of light by employing interference between different optical paths is a useful method for the determination of length or density with very high accuracy. This technique is, therefore, frequently used in the broad range of scientific fields (e.g. gravitational astronomy, biology, etc…). In the context of classical mechanics, the accuracy of the measurement is governed by the classical nature of light; the intensity of light itself. In the context of quantum mechanics, there have been possibilities to overcome this classical limit by using entangled state of several photons, which is a pure quantum state.
We have demonstrated the generation of the 4-photon entangled state and have developed a very stable home-built interferometer. Moreover, our phase measurement of light by using this 4-photon entangled state and the interferometer showed high-sensitivity beating the classical limit.
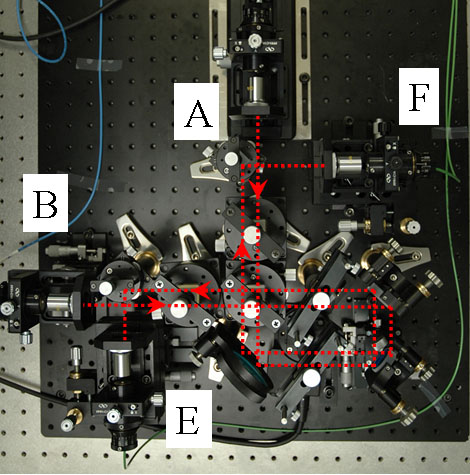
Fig. 2: Experimental setup.
[1] T. Nagata, R. Okamoto, J. O’Brien, S. Takeuchi and K. Sasaki, “Beating the Standard Quantum Limit with Four Entangled Photons”, Science, 316, 726-729 (2007).
Quantum entanglement
Quantum entangled state is a superposition of two or more quantum states, which has particular correlation between different systems. Polarization of a single photon acts as a two-level quantum system (qubit), so that it makes a two-photon two-qubit entangled state. This type of entangled state has been extensively investigated and utilized in many experiments such as quantum cryptography and quantum computation.
Similarly, two photons existing together in the same mode should act as a three-level quantum system (qutrit), although it had never been proved experimentally. We have conducted a proof-of-principle experiment of four-photon two-qutrit entangled state and have succeeded in distinguishing a two-qutrit entangled state from a pair of two-qubit entangled states [1].

Fig. 3: Experimental setup
[1] K. Tsujino, H. F. Hofmann, S. Takeuchi and K. Sasaki, Distinguishing genuine entangled twophoton-polarization states from independently generated pairs of entangled photons, Phys. Rev. Lett. 92, 153602/1-153602/4 (2004).
Single photon source and photon detector
Single photon sources emitting a single photon on demand and photon detectors that can detect photons with 100% efficiency are key components in quantum information technology.
We have proposed and demonstrated a novel technique that can prepare a single photon state. This technique is based on photonic device that always emits two photons in a pair and can find either of these two photons on the basis of the detecting occurrence of the other one [1,2].
We have also developed an ultimate photon-counting system having about 90% quantum efficiency [3, 4].
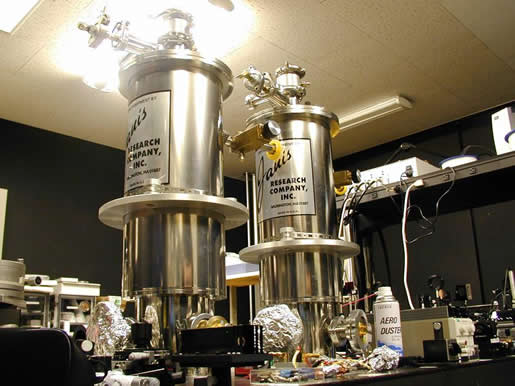
Fig. 4: High-quantum-efficiency single-photon counting system
[1] S. Takeuchi, R. Okamoto and K. Sasaki, “High-yield single-photon source using gated spontaneous parametric downconversion”, Appl. Opt. 43, 5708-5711 (2004).
[2] R. Okamoto, S. Takeuchi and K. Sasaki, “Detailed analysis of a single-photon source using gated spontaneous parametric downconversion”, Journal of the Optical Society of America B , 22, 2393-2401(2005)
[3] S. Takeuchi, J. Kim, Y. Yamamoto, and H. H. Hogue, “Development of a high-quantum-efficiency single-photon counting system”, Appl. Phys. Lett. 74, 1063-1065 (1998)
[4] J. Kim, S. Takeuchi, Y. Yamamoto, and H. H. Hogue, “Multiphoton detection using visible light photon counter”, Appl. Phys. Lett. 74, 902-904 (1998)
Quantum cryptography
The security of traditional cryptography currently used relies on the computational difficulty of certain mathematical functions. It is, however, pointed out that a quantum computer could efficiently solve this type of problems and would break many of the cryptographic systems in use today.
Quantum cryptography, which has the advantage of being able to detect eavesdropping on communication channels, is expected to be an absolutely unbreakable cryptography, because its security is guaranteed by a fundamental physical law. To realize a quantum cryptography communication system, a single photon source is required. If there are more than two photons instead of a single photon, eavesdropper can pick up photons and decipher messages
We have succeeded in constructing a single photon source with the probability of two photon emission less than 0.01%. We have also developed a quantum cryptography communication system by using this high quality single photon source and have realized a proof-of-principle experiment of quantum cryptography system with single photon source over 80km [1,2]. * This is a collaborative work with Mitsubishi Electric.
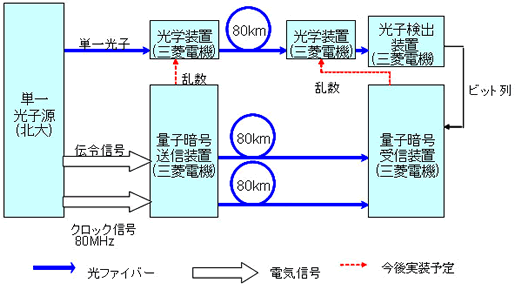
Fig. 5: Quantum Cryptography System
[1] A. Soujaeff, S. Takeuchi, K. Sasaki, T. Hasegawa and M. Matsui, “Heralded single photon source at 1550 nm from pulsed parametric down conversion”, J. Mod. Opt. 54, 467-475 (2007).
[2] A. Soujaeff, T. Nishioka, T. Hasegawa, S. Takeuchi, T. Tsurumaru, K. Sasaki and M. Matsui, “Quantum key distribution at 1550 nm using a pulse heralded single photon source”, Opt. Exp. 15, 726-734 (2007).
pagetopNanophotonics & optical micro-resonator
Nano-optical fiber and solid-state microsphere resonator
Nano-optical fiber possessing a very small diameter comparable to optical wavelength is coupled to solid-state silica microsphere whose size is 10-100 um. This taper-fiber-coupled microsphere resonator may work as a quantum phase gate (QPG), when we embed atoms and quantum dots into the resonator. The solid-state QPG will work with much higher efficiency than conventional QPGs. We believe that the solid-state QPG is a key to the realization of quantum information technology.
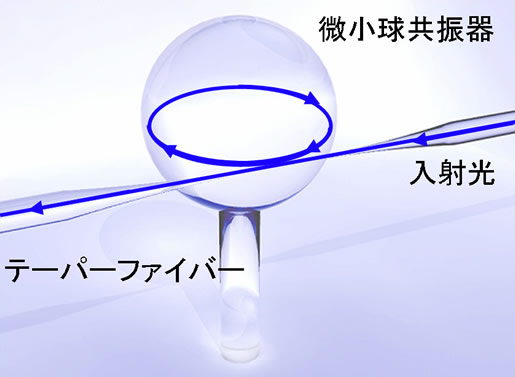
[1] A. Chiba, H. Fujiwara, J. Hotta, S. Takeuchi and K. Sasaki, “Resonant frequency control of a microspherical cavity by temperature adjustment”, Jpn. J. Appl. Phys. 43, 6138-6141 (2004).
[2] A. Chiba, H. Fujiwara, J. Hotta, S. Takeuchi and K. Sasaki, “Fano resonance in a multimode tapered fiber coupled with a microspherical cavity”, Appl. Phys. Lett. 86, 261106/1-3 (2005).
[3] H. Konishi, H. Fujiwara, S. Takeuchi and K. Sasaki, “Polarization-discriminated spectra of a fiber-microsphere system”, Appl. Phys. Lett. 89, 121107/1-3
Generation of non-classical light from a single molecule or single quantum dot.
Semiconductor quantum dots (QDs) having their size of 100-101 nm show large optical nonlinearity and atom-like emission. In particular, core-shell-type CdSe/ZnS QDs have promising optical characters for device applications. Emission wavelength from these dots can be controlled by changing their size, for example. Their emission also shows anti-bunting behavior at room temperature. We use this type of single QDs for the development of highly efficient single photon sources and nonlinear quantum devices.
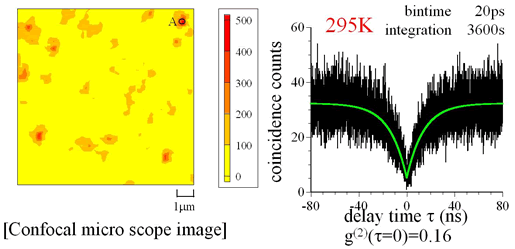
Controlling the emissions from atoms in microsphere resonator
The taper fiber allows us to efficiently inject light into the microsphere resonator, in which very high quality factor is achieved. Using this taper-fiber-coupled microsphere resonator, we demonstrated the lasing of Er & P sol-gel glass layer on the microsphere. The thickness of the gain layer is ultimately thin on the order of ~ 100 nm. Furthermore, we have shown that the coupling efficiency of spontaneous emission into the modes of microsphere cavity can be greatly enhanced by controlling the depth of the gain layer in the sphere.
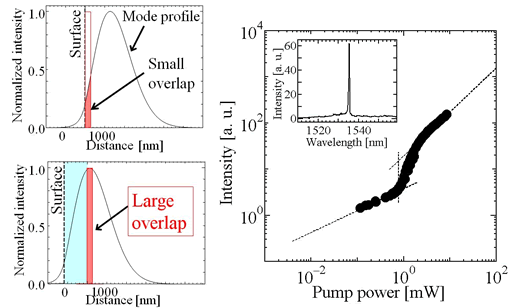
[1] Hideaki Takashima, Hideki Fujiwara, Shigeki Takeuchi, Keiji Sasaki, and Masahide Takahashi, “Control of spontaneous emission coupling factor in fiber-coupled microsphere resonators”, Appl. Phys. Lett. 92, 071115 (2008).
[2] Hideaki Takashima, Hideki Fujiwara, Shigeki Takeuchi, Keiji Sasaki, and Masahide Takahashi, “Fiber-microsphere laser with a submicrometer sol-gel silica glass layer codoped with erbium, aluminum, and phosphorus”, Appl. Phys. Lett. 90, 101103 (2007).
pagetop